Early AGB Evolution
Stellar Evolution Tutorial (Demo Version)
-
Introduction
- The "Asymptotic Giant Branch" is so-named because for some
stars the evolutionary track up the giant branch, for the second
time, approaches the first giant branch almost asymptotically.
It used to be called the "second giant branch" which is perhaps
a better name, rather than relying on a morphology which is not
always present or obvious. In any event, the AGB evolution is
divided into two phases: the early-AGB (E-AGB) and the thermally
pulsing AGB (TP-AGB). The onset of thermal pulses is the dividing
line between these phases.
- The graphs and models shown in this section are from the
evolution of a 5Msun model with Z=0.02.
-
E-AGB
- The second dredge-up phase
is actually
a part of the E-AGB, but was discussed earlier. We shall only
briefly discuss the E-AGB again, as we shall concentrate
on the thermally pulsing phase. Note that the second dredge-up
does not occur for all masses, but only for masses above about 4 Msun,
although this number depends on the composition slightly.
- Following core He exhaustion, the He shell is established and
as it moves through the He profile the high luminosity generated
causes the star to expand, and the H shell is extinguished (or
nearly so). Thus the continued expansion causes the outer layers
to cool, and the opacity rises, causing convection to dominate
as the energy transport mechanism. Hence the second dredge-up
phase is initiated. The graph below shows the inner edge of the
convective envelope moving inward, through the position of the
H-shell (defining the H-exhausted core). All the time the He
shell is moving outward, also. The shells get very close together,
as shown in the diagram.
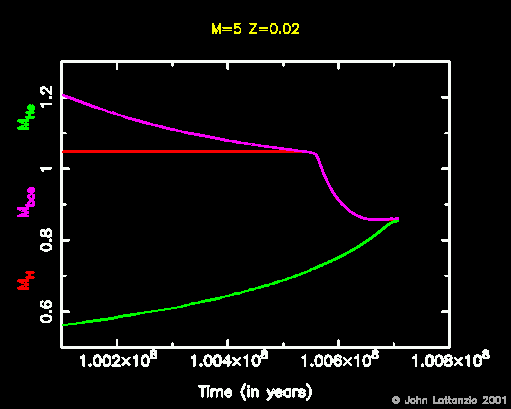
- The next movie (
horizontal and vertical )
shows the H and He profiles versus time during the times shown in the above
diagram. A grey line moves across the diagram above to indicate the time for
which the abundance profiles are being displayed. Note the He shell advancing
outward due to burning, and the H shell being pushed inward by the dredge-up.
The final result is that the H ad He shells are very very close
together!
- The CO core: core He burning initially produces C-12 from the
triple-alpha reactions, and then once there is some C-12 present the
C12-(alpha,gamma)O-16 reaction produces some O-16, as we saw in the
earlier evolutionary phases. But shell
He burning is another matter. By the time there is some C-12 built up there
is very little He left to form O-16. A convective core keeps mixing in
fresh He but a radiative shell does not. Hence the main product of shell
burning is C-12 rather than O-16. The C ad O abundances during the E-AGB
phase are shown in the next movie.
- The next movie shows
the luminosity profiles during the E-AGB phase, including second dredge-up.
You can clearly see the rise in the luminosity. Also, the line is colour
coded according to the H and He abundance. The cyan part shows the region
of variable H abundance, which is usually the H burning shell. But when
the second dredge-up begins there is no region of variable H abundance!
The H content drops from the surface value to zero at a discontinuity.
Hence the cyan points disappear during second dredge-up!
However, then we have two very slightly different shades of blue next to
each other, so these movies will be re-made (see the
full version ) now that this feature is so clear!
Nevertheless, it is very instructive: you can see (if you look
carefully!) the join between these two shades of blue move inward with
time: this is the second dredge-up. All the while, of course, the He shell
is strengthening and the luminosity is increasing. The He shell is shown
in magenta and it is clear that this is providing all of the luminosity
during this phase. At the end of the second dredge-up the H shell is
re-ignited and the last frame shows the cyan H shell in place again,
and also shows that it provides most of the star's luminosity now that
the He shell has reached its new position. The picture below shows all
the L profiles superposed on each other.
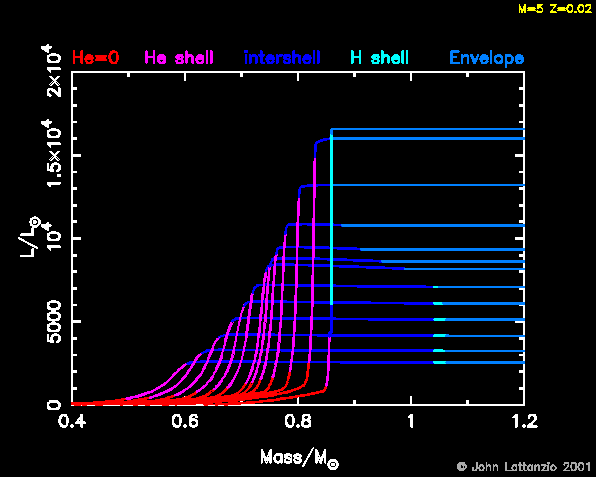
- Next we show the temperature.
There is a lot of information here, too. Firstly, notice how the
relatively flat profile initially turns into the classic core-envelope
structure, with a very flat (isothermal) core and a very steep drop at
the edge of the core. Again, you can see the cyan region (the H shell)
disappear during dredge-up. Just prior to this you can see it cool, then
it moves inward in mass as a result of the dredge-up. Finally, the shell
is re-established and the star has a double shell-source structure: a
He-shell (shown in magenta) and a H-shell (shown in cyan).
- The radius shows
the expansion also. Watch the H-shell being propelled outward by the
energy liberated by the He shell. Then the second dredge-up moves it
inward in mass (but at almost constant radius!) while the He-shell moves
outward and gets thinner. Eventually we have a very compact CO core (at
a radius of about 0.01Rsun, and then the He and H shell at almost the
same mass, and also very close together in radius.
- The density shows
a predictable behaviour, again displaying the core-envelope structure
and the close proximity of the H ad He shells.
-
The Thermally Pulsing-AGB
- We now move toward the first thermal pulse. The two shells
are geometrically thin and very close together, and this, combined with
the high temperature dependence of the helium burning reactions, is
thermally unstable. The output of the shell begins to oscillate as show
below. Then a genuine thermal pulse develops, with the helium luminosity
reaching up to a few times 10^5Lsun.
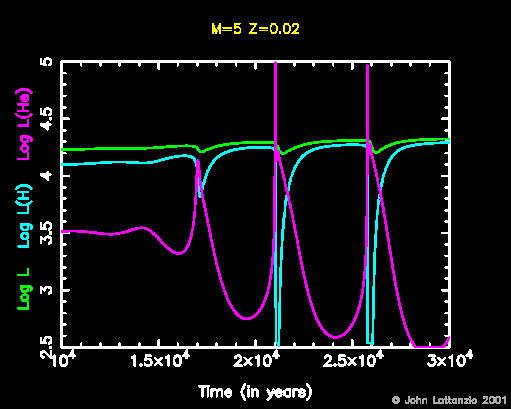
- Of course, this energy causes the star to expand, and the H shell is
propelled outward to lower temperatures and densities so it is almost
extinguished. Note that the total surface luminosity hardly changes,
however!
- It is instructive to look at the time variation of some critical mass
positions in the star. The figure below shows the inner edge of the convective
envelope as well a the position of the H-shell (defining the H-exhausted
core) as well as the He-shell (defining the He-exhausted core).

- The cyan line shows the inner edge of the convective envelope.
This will show dredge-up in later pulses, but not for these earlier ones.
The red line is the center of the H-shell, which is equivalent to the edge
of the H-exhausted core. Finally the green line shows the edge of the
CO-core, or the position of the He-shell. Note that the He-shell moves
outward primarily during a pulse, rather than between pulses. On the
contrary, the H-shell moves out3ward during the interpulse phase mostly,
and is essentially stationary during the (brief time of the) pulse.
- Here ( horizontal and
vertical ) are the H and He
profiles during the early pulses. Note the alternate movement mentioned
above: the H-shell moves outward mostly between pulses, when the star is
powered by H-burning. But at the pulse, the He-shell is
active and the He-shell position moves outward (this is all in mass, of
course!).
- Next is the ( horizontal
and vertical ) He, C-12 and O-16
profiles during the early pulses. Note that the outer edge of the red line
(the H-shell) moves outward during the time between pulses. Then, at the
pulse, the inner edge of the red line (where the He is destroyed, thus
indicating the position of the He-shell) catches up. In fact, the inner
edge of the red line corresponds also to the production of C-12, whose
profile is shown in green, and the small amount of O-16 is show in blue.
These all move pretty much as one, during the pulses, which is to say,
during the time of active He burning. )
- Here are the luminosity profiles (
horizontal and vertical ) during
the early pulses. The profile is colour-coded to show whether it is the H or
He shell that is producing the energy. Initially, the H shell is clearly
visible (cyan part and produces most of the energy. You can see the
oscillations of the He-shell (magenta) as the first major pulse approaches.
Note that the H-shell is extinguished during the pulse: the energy
generated by the He-shell is very large, and goes into expanding the star.
You can see the region of absorption: it is where the luminosity
decreases with mass, moving outward. Note that despite the contortions
of the L profile, the surface luminosity (green line i second frame, or
very nearly the right-most value in the L-profile frame) is almost
unchanged. )
- Using the same colour-coding, we show here the T profiles
( horizontal and
vertical ) during the early
pulses. The second frame shows the
time-variation of the temperature at a representative position in the H and
He-shells. Note that their behaviour is opposite of each other! During a
pulse, the He-shell gets hotter but the H-shell cools (due to the expansion
powered by the energy from the He-shell). )
- Closely related to the previous figure is the behaviour of the
density in the shells. In this case we see the expansion of the star is
evidenced in the decrease in density.
- The last in this series shows the position of the two shells, in
radius. For these pictures we have picked a point near the bottom of the
H-shell (where the H abundance is about 0.05 typically) so that we can
display both shells on the same axes. The He shell position is closer to
the centre (i.e. to a He mass-fraction of about 0.5). Again, here we see
the dramatic expansion of the H-shell, being propelled outward in radius
by a factor of 2!
- The instability that we have seen develop in the above, continues
to recur and grows in strength during the AGB evolution. Here are the first
few pulses for the 5Msun model with Z=0.02. Note that the Helium luminosity
grows to
10^8 Lsun very quickly.
- The figure below shows the position of the H and He-shell,
as well as the convective4 envelope, during the same time as the above
diagram shows the growth of the He instability. With each pulse, the
expansion gets stronger
and eventually the convection reaches in beyond the (now extinct) H-shell.
This is the "third dredge-up" and is clearly seen below. The green line
(the inner edge of the convective envelope) moves into the red line. This
mixes C-12 to the surface (more on this later) and it also pushes inward
the H/He discontinuity. Hence when the H-shell is re-ignited it does so at
a smaller mass-value than before. The depth of the dredge-up typically
grows with each pulse. This depth is measure by the "dredge-up parameter
lambda", to be discussed below.
-
Anatomy of a Thermal Pulse
- We will now look at one pulse in more detail. We will then look at
two consecutive pulses, to see how they interact and overlap.
- Here is a close-up of the luminosity sources during the pulse.
Note that the time-scale for this picture is only 150 years. Once the He
luminosity becomes large, the H-shell is extinguished, and its output drops
to almost zero. The star acts as a luminosity thermostat, however, and the
total output from the surface is almost constant!
On the same scale, we show next the important mass boundaries in
the star. Convective regions are shown in blue: these are the convective
envelope and the inter shell convective zone. When the He-shell "flashes"
it dumps more energy than can be carried by radiation, and a convective
zone develops. The abundance in this convective zone reflects the products
of the He burning reactions its about 25% C-12 and 75% He-4. This material
is pushed almost all the way to the H-shell. The large expansion caused by
the input of energy from the He-shell, now causes convection to grow inward
as the opacity rises at lower temperatures. This the convective envelope
reaches across the extinct H-shell and into what was the intershell
convective zone. Thus the convection, termed "dredge-up", succeeds in mixing
material to the surface that is enriched in C-12. There is much more
nucleosynthesis going on, and this will be discussed
later.
- This movie shows the He
profiles during the pulse. At the peak we see the flat profile corresponding
to the
rapid mixing in the convective zone. After the peak, the convective envelope
moves inward and mixes up the freshly made He-4 to the surface. Note that
the H0shell is extinguished prior to the dredge-up.
- Here we show the same He profiles
( horizontal and
vertical ) together with the
convective zones, for extra clarity.)
- This movie is similar, but
it shows the C-profile as well as the He-4 profile. Obviously, C-12 is the
product of He-4 burning, and this shows the production of C-12 and then its
mixing to the surface by the third dredge-up.
- Here ( horizontal and
vertical ) is the L profile during
the pulse. This clearly shows the He-shell providing the energy as well as
the expansion from the energy this produces.
- Here are the T profiles (
horizontal and vertical ) and
the time-dependence of the temperature in the shells. We have picked one
position for the H-shell in the second frame, but for the He-shell we have a
typical point at the top, the middle and the bottom of the He-shell. Note
how the lines cross-over! This is because there is a temperature inversion:
the temperature is not monotonic through the He-shell during a pulse. You
can see this clearly in the first frame which shows the T distribution vs
mass.
- This is the same, but the second frame is the mass zones and
convection is shown in red (
horizontal and vertical
). Likewise, radiative zones are shown in green on the first frame
and convective zones are red, So as the grey line, indicating the current
time, moves into a red zone (on the second frame) then the corresponding
part of the T profile should also become red. This is a nice way of seeing
the temperature of the intershell convective zone. As you can see, it
reaches temperatures approaching 10^8.5K. One can also see the dredge-up,
in red again.
- Here ( horizontal and
vertical ) are the density
profiles. Again, there is only one position shown for the H-shell, but
three positions within the He-shell.
- And here ( horizontal and
vertical ) are the radial positions
of the same mass positions. The expansion is clearly visible here.
-
Two Consecutive Thermal Pulses
- Now we look at two consecutive thermal pulses. There is
interesting interaction between them. This is especially important for the
nucleosynthesis. Naturally, some of the ideas from
above are repeated here.
- Here are the main luminosity sources: the H and He-shells.
Note that H powers the star throughout most of the evolution, with brief
bursts from He at the pulses. The sources alternate in importance, with H
providing most of the energy in time, but He catching up with short but
strong bursts! The surface value is hardly changed....
The luminosity sources are reflected in the motion of the H- and
He-shells in mass, as shown below. The dredge-up is clearly seen. Note that
the intershell convective zones are just thin vertical lines on this scale.
- An important parameter is the so-called dredge-up parameter,
lambda. This is defined as the ratio between the amount of material dredged
to the surface following a pulse, Delta M_dredge, and the amount of mass
processed between two pulses by the H-shell, Delta M_H. This is shown
below.
- Here ( horizontal
and vertical ) are abundance
profiles for He, C-12 and O-16. You can see the alternate advance of the H
and He-shells, the intershell convection, and the dredge-up.
- Here are the L profiles (
horizontal and vertical )
- Here are the T profiles and shell values
( horizontal and
vertical. ) Note the temperature
inversion during the pulse.
- Here is density profiles and shell values
( horizontal and
vertical )
- Here are the radial positions vs mass, and the radial positions of
the shells. Note how the H-shell is "flicked" outward by the He shell flashing.
( horizontal and
vertical )
Return to the stellar evolution page.
Go to the nucleosynthesis page.